DIII-D National Fusion Facility
Research
What is fusion?
Fusion is the process that powers the stars, including our Sun! At the core of every star, you find a hot, dense soup of hydrogen atoms. The high temperatures present in the core cause neutral hydrogen atoms to break apart, separating into positively-charged nuclei and negatively-charged electrons that form a plasma – also referred to as the 4th state of matter. While two positively charged nuclei will repel each other due to both being positively charged (akin to two north poles of magnets repelling each other), nuclei with enough energy, such as those in the Sun’s core, can overcome this repulsion. Once they are close enough, the two nuclei can begin to attract each other and then fuse into a larger, more stable nucleus. During this fusion reaction, a small amount of mass is converted into a large amount of energy. The fused nucleus will then go on to fuse with other light nuclei (like helium), resulting in many fusion reactions in the Sun per second. The enormous amount of energy released in the Sun through fusion then goes on to sustain life here on Earth!
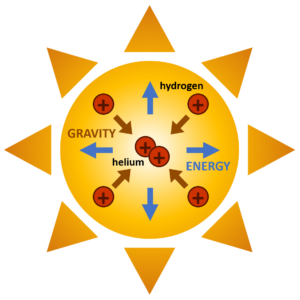
Why do we care about fusion?
Simply put, fusion has the potential to serve as the basis of a new and extremely advantageous source of clean and reliable energy for humanity. Whether it’s growing the food we eat, heating and cooling our homes, or powering the electronic devices we use for work, communication, and entertainment, every aspect of our modern society relies on energy. In recent years, global power consumption has risen above 20 TW (electricity used per unit time); for reference, the average power output of the Hoover Dam is 0.001 TW! [1] The global demand for energy will only continue to increase as the population grows and average quality of life increases.
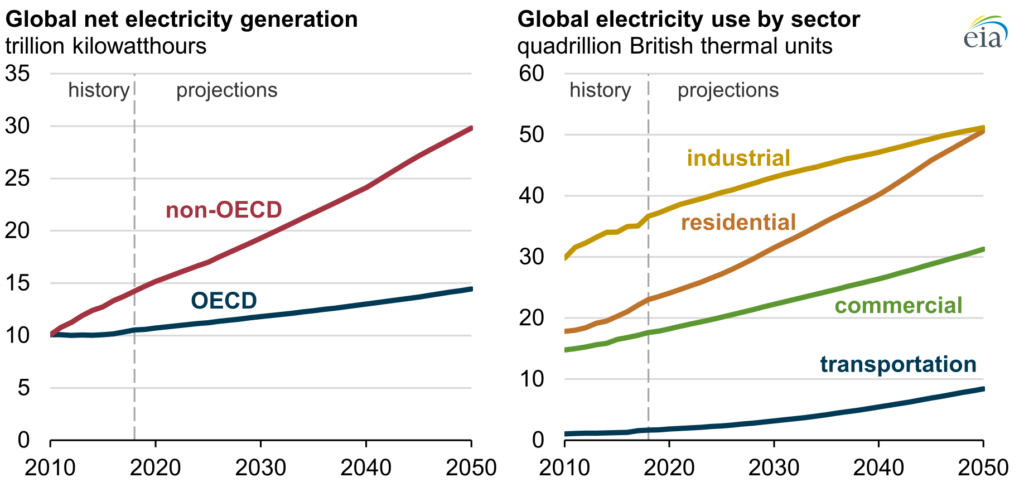
The power that we derive from fossil fuels has helped create the modern world that we know today. While these energy resources are readily available and inexpensive, they are also nonrenewable and detrimental to our climate, with fossil fuel consumption resulting in the release of enormous amounts of pollution and unnatural changes to our climate. The Intergovernmental Panel on Climate Change Sixth Assessment Report states that “…human-induced climate change has caused widespread adverse impacts to nature and people” and highlights the importance of “limiting global warming to 1.5°C if we are to achieve a fair, equitable and sustainable world”. [2]
Several clean energy solutions are being developed to reduce our dependence on fossil fuels, which will limit future detrimental impacts on the world. Fusion is being developed as one of these solutions to provide large amounts of clean, reliable energy in the future because it:
- Does not directly produce any pollution or greenhouse gases.
- Uses fuel that comes from water (including seawater) and lithium (the same element used in your phone battery). Scientists estimate that there is enough fuel on Earth to power the world using fusion energy for up to millions of years.
- Generates a large amount of energy from a very small amount of fuel. The hydrogen in just three water bottles could be used in a fusion reaction to produce the same amount of energy as that released by a combustion reaction performed with the amount of coal carried in 80 train cars. Using the famous equation E=mc^2, we estimate that one soda can of hydrogen atoms could produce enough energy through fusion to power a home for 3 days! [3]
- Can provide a constant, uninterrupted source of power 24 hours per day, 7 days per week.
- Does not produce any long-lived radioactive waste and is incapable of producing a runaway nuclear reaction.
Developing this new technology also provides incredible opportunities to spark American innovation, create a new field with diverse job opportunities, and improve domestic energy security.
How do we generate energy from fusion on Earth?
Generating star power here on Earth is difficult! We do not have the enormous gravitational force of the Sun to generate the high pressures needed to keep energetic particles from escaping the reaction. Instead, fusion on Earth is achieved using one of two general methods for heating, compressing, and confining the fuel: inertial confinement and magnetic confinement.
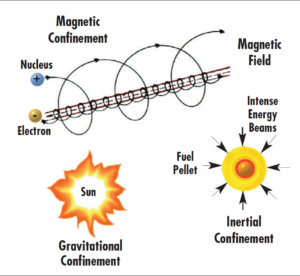
Inertial confinement involves compressing fuel to a fraction of its original volume using laser beams or shockwaves. Sudden increases in temperature and pressure create ideal conditions for fusion to occur for a few billionths of a second. Necessarily then, generating steady power from inertial confinement fusion requires individual implosions to be generated at a rapid pace. Learn more here.
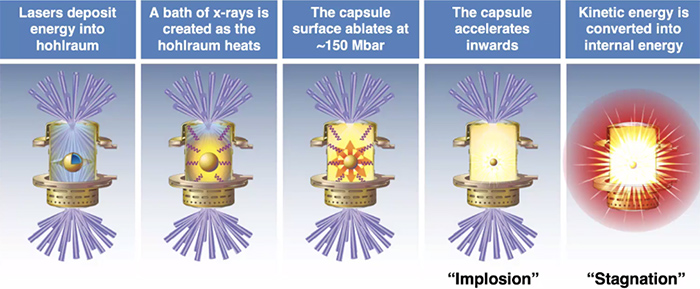
Magnetic confinement involves heating fuel to millions of degrees to generate a plasma and then compressing and controlling the hot plasma using magnetic fields to create conditions favorable for fusion to occur over multiple seconds. The DIII-D National Fusion Facility utilizes magnetic confinement to create fusion reactions and study the physics principles of a magnetic fusion reactor. DIII-D is a tokamak, which is a toroidally-shaped chamber (like a doughnut) surrounded with magnetic coils. Once fuel is injected or pumped into the chamber, the coils are used to create toroidal (long way around the doughnut) and poloidal (short way around the doughnut) magnetic fields that confine the plasma and create the conditions for fusion to occur.
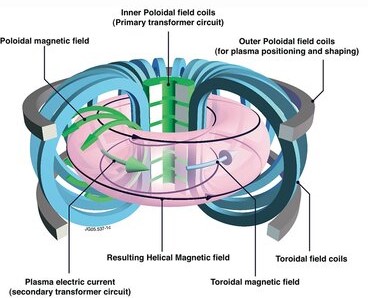
The major challenge for fusion energy on Earth is deriving more energy from fusion reactions than it takes to initiate and sustain these reactions. The physics of the fusion process determines the required temperature for maximizing fusion power (100-200 million degrees Celsius for the type of fusion being pursued at DIII-D). The density and confinement (a measure of how well the plasma can retain heat and particles) then set how much fusion power will be produced and whether that power will exceed the minimum power needed to sustain the reaction. A large portion of the research at DIII-D is focused on overcoming the challenges that limit density and confinement to support the goal of developing a future fusion device that is a net source of energy.
Regardless of how fusion energy is generated, it always requires three ingredients (known as the “triple product”):
- High temperature
- High pressure
- Long confinement time

While fusion energy can be produced from many different types of reactions, scientists primarily focus on one specific reaction, referred to as deuterium-tritium fusion (“DT fusion,” for short). Two isotopes of hydrogen, deuterium (with one additional neutron) and tritium (with two additional neutrons), are fused together at high temperatures and pressures, forming a helium nucleus and a neutron. While tritium is radioactive, its half-life is much shorter than that of some long-lived radioactive isotopes in spent nuclear fuel used in fission reactors.
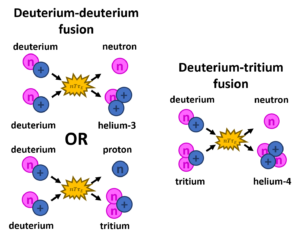
DT fusion requires the lowest temperatures to initiate the reaction and is, therefore, the focus of much of the world’s fusion research. While deuterium can be found readily in water (~0.02% of all water molecules contain deuterium atoms), tritium does not occur naturally in significant amounts. To overcome this, scientists have proposed constructing a liner made of lithium that wraps around the interior of a fusion reactor (referred to as a “blanket”), which will produce tritium when struck by the neutrons generated during the DT fusion reaction.
Due to the low supply and radioactivity of tritium, as well as the immature technology for blankets, the DIII-D tokamak focuses on the deuterium-deuterium fusion reaction (“DD fusion,” for short) rather than DT fusion. While this reaction is more difficult to sustain and yields a lower energy output, scientists are still able to study much of the underlying physics that will inform the design and operation of a future fusion power plant performing DT fusion. The lack of radioactive tritium in the vessel and at the facility also significantly expands personnel access to the machine, enabling continuous upgrades to the vessel to support an agile, modern research program.
Where is fusion energy being studied?
Our team at the DIII-D National Fusion Facility makes fusion energy every day! DIII-D is the largest magnetic confinement facility in the United States and has been enabling foundational research on the scientific basis of fusion energy for over 30 years. Learn more about DIII-D’s history here. Regular upgrades to the facility allow the team to continuously stay on the cutting edge of the field, informing the design and operation of future fusion experimental facilities and power plants.
The National Spherical Torus Experiment Upgrade is the other major magnetic confinement facility in the U.S., located at Princeton Plasma Physics Laboratory. Learn more about this facility here.
The U.S. Federal Government is also a partner in the ITER project, which is the largest fusion experiment in the world. ITER is a magnetic confinement facility being constructed in southern France. Over 30 nations are contributing to this exciting project. Learn more here.
Outside of these major facilities, many other publicly- and privately-funded institutions operate tokamaks, as well as other devices, to advance the field towards the realization of a functioning power plant. Small-scale university experiments and advanced computational physics research also play major roles in studying related plasma physics and enabling complex computer simulations of plasma behavior. University degree programs in plasma physics, nuclear engineering, and related disciplines educate the next generation of the fusion workforce. Learn more about the U.S. fusion energy landscape here.
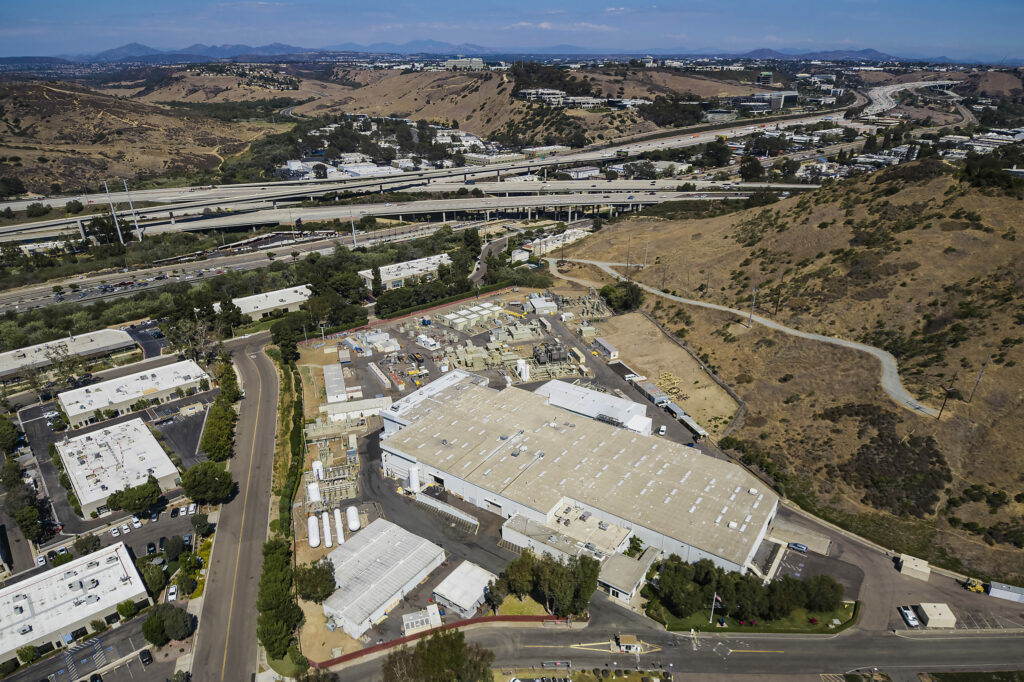
Who studies fusion energy at DIII-D?
Our research team at DIII-D consists of hundreds of scientists, engineers, and students supported by many technicians, administrators, and specialists. DIII-D team members can be found both on-site and around the world. The wide array of expertise and knowledge among team members facilitates a dynamic and ambitious research program. Learn more about the team here.
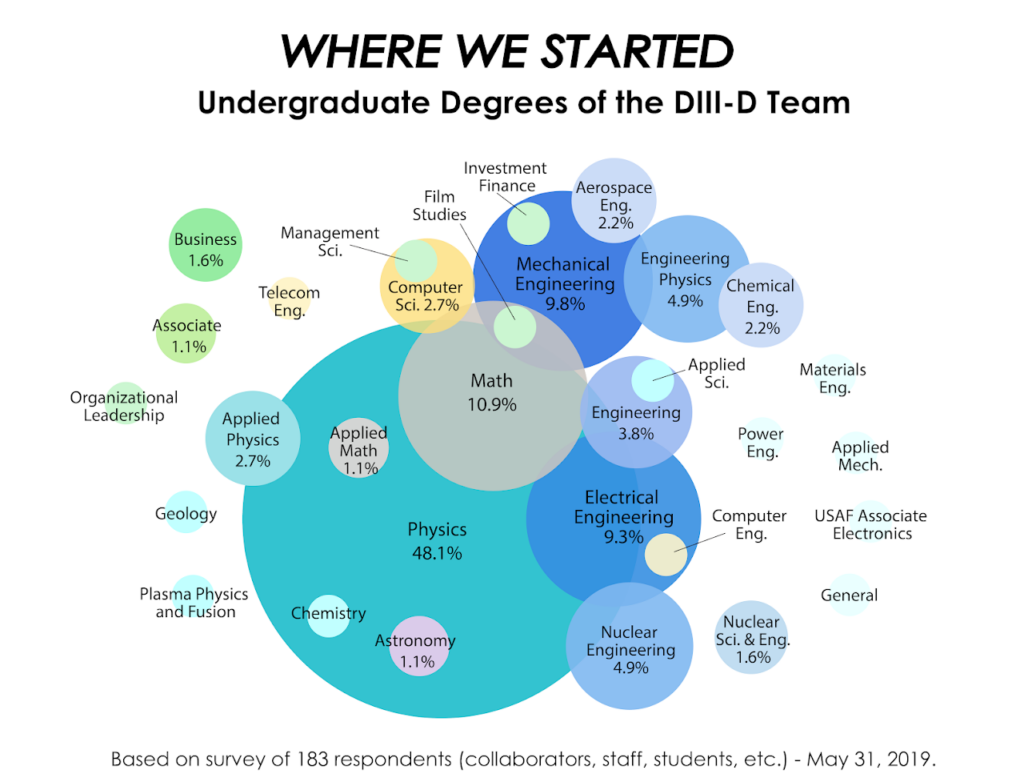
When will we have fusion power plants?
As described above, the primary challenge in fusion energy is generating more energy from the reaction than was used to initiate the reaction. Encouragingly, scientific and technological developments over the years have brought us closer to this threshold, known as “breakeven.” Beyond the point of breakeven, the conditions needed for fusion to occur can start to be sustained by the output of the fusion reaction itself. However, further work will be needed to steer the plasma to a state where the gain above breakeven is large enough to support the practical production of electrical power. The ITER project aims to produce 10 times more power from the reaction than what is absorbed by the plasma. At DIII-D, our team continues to answer important physics questions to support the achievement of this goal, thereby informing the design and operation of a successful fusion power plant.
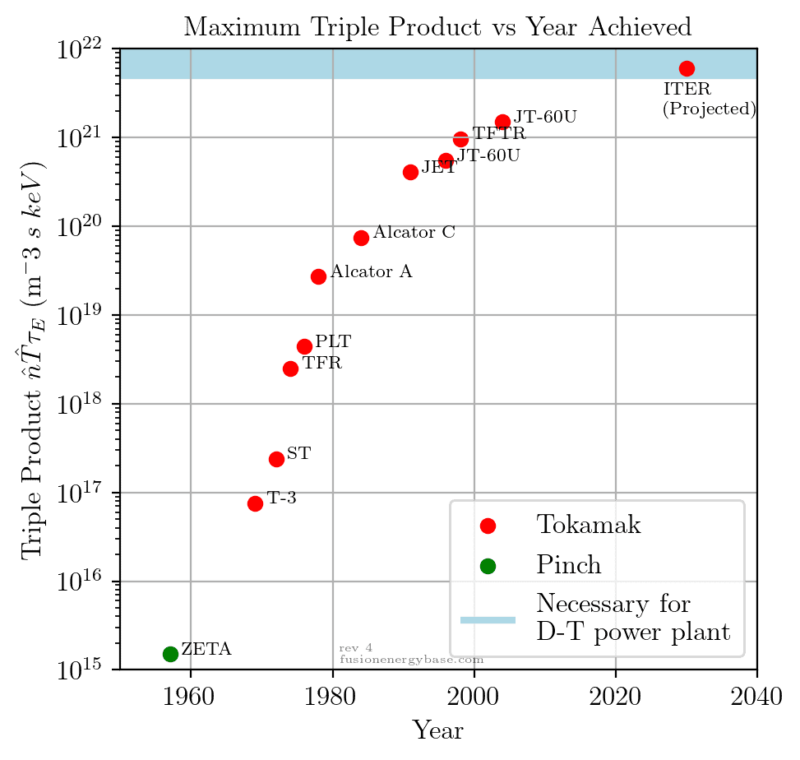
Recent reports authored by members of the U.S. fusion community lay out a timeline for a “pilot plant” – a demonstration device that would precede a commercial-scale power plant. The reports “Bringing Fusion to the U.S. Grid” by the National Academies of Science, Engineering, and Medicine and “A Community Plan for Fusion Energy and Discovery Plasma Sciences” by the U.S. fusion and plasma physics community call for the construction and operation of a fusion pilot plant in the 2040s.
Dedicated funding, continued public support, and a diverse and growing workforce are needed to address the large-scale challenge of developing fusion as a clean energy solution for a sustainable future.
Citations
- International Energy Agency, “Electricity Information: Overview,” International Energy Agency, 2021. [Online]. Available: https://www.iea.org/reports/electricity-information-overview/electricity-consumption.
- Intergovernmental Panel on Climate Change, “Climate Change 2022: Impacts, Adaptation and Vulnerability. Contribution of Working Group II to the Sixth Assessment Report of the Intergovernmental Panel on Climate Change,” Cambridge University Press, Cambridge, UK, 2022.
- American Museum of Natural History, “How the Sun Works,” American Museum of Natural History, 2002. [Online]. Available: https://www.amnh.org/exhibitions/einstein/educator-resources/how-the-sun-works.